- Ruhr-Universität Bochum
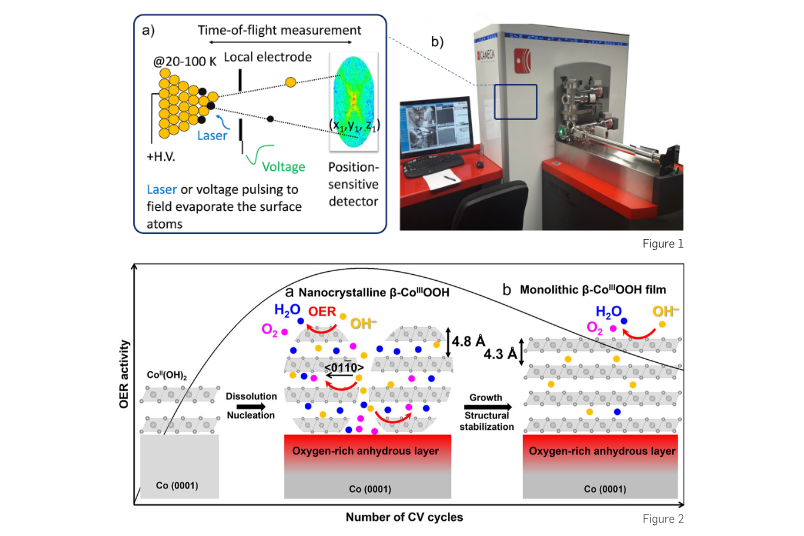
Atomic-scale characterization of cobalt-based electrocatalysts for hydrogen production
With the global population predicted to double by 2070, and with the energy requirements of developing nations increasing significantly, access to affordable and renewable energy is one of the cornerstones of continuing global prosperity and economic growth. Most renewable energy devices and systems use catalyst materials to increase energy conversion rates. The morphology, composition and electronic structure of active sites and chemical species within the topmost atomic layers of electrocatalysts are crucial in the activity and selectivity of electrochemical reactions. In order to make technological promises reality and to achieve meaningful environmental impact, we must fully understand the state of individual atoms within the surface layers of the electrocatalysts in order to link the fabrication, structure and properties. To date, it has not been possible to undertake a full three-dimensional (3D) structural and chemical characterisation of the topmost atomic layers of the electrocatalysts. This has hindered a complete evaluation of the contribution made by individual atoms in this extended zone to the interplay of catalytic activity, selectivity and stability in several important chemical reactions such as the oxygen reduction/evolution reaction (ORR/OER) for water electrolysis.
Thus, there is an urgent need for a new approach to characterise catalyst materials. My group employs atom probe tomography (APT) to resolve individual atoms of electrocatalysts in 3D. APT is a non-routine characterisation technique for catalyst materials. APT, which combines field ion microscopy with mass spectrometry, provides a uniquely powerful insight into the 3D atomic-scale chemistry and structure of materials. APT analysis requires needle-shaped samples with a tip diameter of 30-100 nm. Surface atoms are field-evaporated by a combination of constant voltage and either a high voltage pulse or a laser pulse, as illustrated in Fig. 1. Then the field-evaporated ion is projected on a single-ion-sensitive detector which records its x-y-z coordinates, and the time between the applied pulse and arrival of the ion, allowing a built-in mass-to-charge spectrometer to measure the mass-to-charge ratio. A 3D APT reconstruction can be achieved with individual atoms spatially and chemically resolved.
In our recent work (DOI: 10.1021/acscatal.2c03903), we developed a correlative approach with Professor Kristina Tschulik’s group by combining APT with X-ray photoelectron spectroscopy (XPS) and electrochemical measurements to investigate the temporal evolution of oxidation state, thickness, morphology and elemental distribution of cobalt oxyhydroxides during OER. We reveal that the oxyhydroxide layer with the highest OER activity is 5-6 nm thick β-CoOOH(0001) film, consisting of stacks of nanocrystals; this explains the amorphous characteristic of active species formed on Co-based electrocatalysts observed by operando X-ray-based techniques. The interfaces between the β-CoOOH(0001) nanocrystals accelerate the intercalation of water molecules and hydroxyl groups along β-CoOOH(01‾10) into slabs, enhancing the OER activity. As OER proceeds, the β-CoOOH(0001) nanocrystals grow into a monolithic β-CoOOH(0001) film along with the water deintercalation (Fig. 2). The decreased number of interfaces serving as active regions most likely leads to a slight decrease in the OER activity, although the surface species are highly reversible. Overall, our study unprecedentedly unveils that in situ generated thin β-CoOOH(0001) layer undergoes dynamic morphological and elemental changes along with (de)intercalation of water molecules and hydroxyl groups during OER, which in turn alters OER activity. We demonstrate the strength of our multimodal characterization approach when seeking mechanistic insights into the role of structural and compositional evolution of hydrous oxides in activity during electrocatalytic reactions.